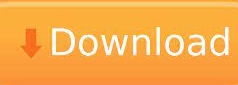

In addition, although a large number of bright green-emitting FPs have been developed, most of them are less photostable than enhanced GFP (EGFP).
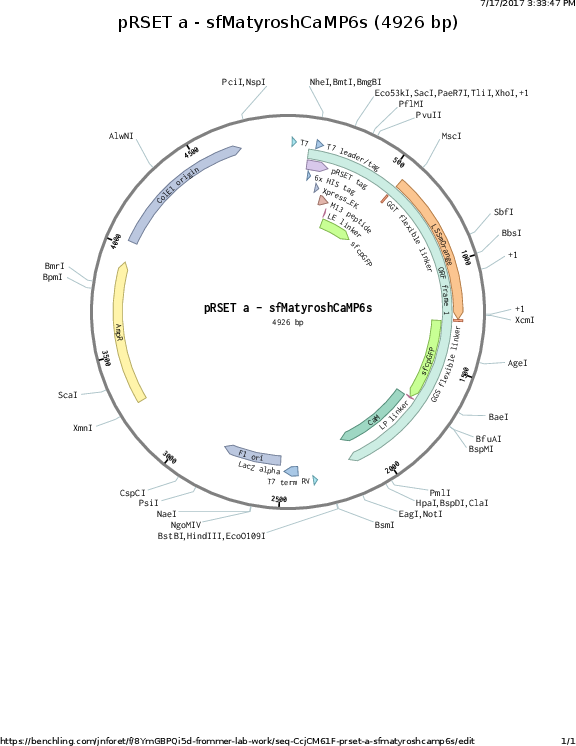
On the other hand, a fast-maturing variant of mKO (mKO2) was developed at the expense of photostability 15. Subsequently, a variant of mOrange (mOrange2) was developed that achieved photostability at the expense of maturation speed 6. mOrange matures faster but photobleaches more easily than mKO. Accordingly, there is a tradeoff between brightness and photostability in FP performance.Ī typical example of this tradeoff can be seen in the evolution of two monomeric orange-emitting FPs, mOrange 13 and mKusabiraOrange (mKO) 14, which were developed independently by two research groups.

In this regard, enhanced O 2 accessibility decreases photostability. However, decomposition of FP chromophores also involves O 2, which reacts in a photochemical reaction with the chromophores that remain in their singlet or triplet excited states 12. The main complication arises from molecular oxygen (O 2), which is required for maturation of chromophores of FPs, and high accessibility to O 2 contributes to the increase in maturation speed and maximal brightness in cells 11. 9), and a recently published photostable yellow-emitting FP, mGold 10.

Exceptions could be observed in the development of stable and bright blue-emitting FPs, such as Azurite 8 and EBFP2 (ref. To date, the development of photostable FPs has nearly always been accompanied by a decrease in brightness 6, 7. Highly photostable FPs are, therefore, needed to enable fast and long super-resolution imaging. Similarly, obtaining a high spatiotemporal resolution to study the fast dynamics of fine subcellular structures requires continuous acquisition of images on a time scale from seconds to minutes 4, 5, which inevitably leads to noticeable FP photobleaching. However, obtaining high signal-to-noise-ratio images from samples with low-level expression requires strong excitation light, which often causes severe photobleaching. For most applications, as low as possible expression levels of an FP-tagged protein are preferable to minimize the perturbation of the system under observation 3. Over the past few decades, owing to improvements in gene transfer and protein-targeting techniques, researchers have succeeded in labeling subcellular components, such as organelles and the cytoskeleton, with fluorescent proteins (FPs), enabling efficient observation of these structures in living cells and organisms 1, 2. StayGold will substantially reduce the limitations imposed by photobleaching, especially in live cell or volumetric imaging. As StayGold is a dimer, we created a tandem dimer version that allowed us to observe the dynamics of microtubules and the excitatory post-synaptic density in neurons. Using StayGold fusions and SIM, we also imaged the dynamics of mitochondrial fusion and fission and mapped the viral spike proteins in fixed cells infected with severe acute respiratory syndrome coronavirus 2. We used StayGold to image the dynamics of the endoplasmic reticulum (ER) with high spatiotemporal resolution over several minutes using structured illumination microscopy (SIM) and observed substantially less photobleaching than with a GFP variant optimized for stability in the ER. StayGold is over one order of magnitude more photostable than any currently available fluorescent protein and has a cellular brightness similar to mNeonGreen. Here we present StayGold, a green fluorescent protein (GFP) derived from the jellyfish Cytaeis uchidae. The low photostability of fluorescent proteins is a limiting factor in many applications of fluorescence microscopy.
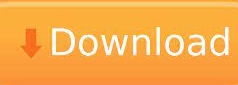